Pioneering progress in cancer treatments
From its research reactor to revolutionary therapies, Mizzou’s innovations are shaping the future of precision medicine.
Contact: Uriah Orland, uriah.orland@missouri.edu, 573-884-5139
Bringing a new medicine to market can be a long journey, but the potential to save lives makes it worthwhile.
Twenty years ago, the University of Missouri helped research and develop a little-known radioisotope called lutetium-177 (Lu-177). That innovation laid the foundation for clinical trials and Food and Drug Administration (FDA) approvals for a new class of drugs known as radiopharmaceuticals, resulting in thousands of successful cancer treatments.
More than 2,000 clinical trials for cancer treatments using radioisotopes like Lu-177 are now underway. In this burgeoning field, Mizzou is the essential first stop — because the University of Missouri Research Reactor (MURR) is the only supplier in the United States creating the part of these drugs that destroys cancer cells while sparing healthy ones.
“Our research reactor has been at the forefront of the development and production of radioisotopes that are saving lives every day,” said Matt Sanford, MURR’s executive director. “MURR’s innovative design and year-round operating cycle allow us to reliably produce the active pharmaceutical ingredients in multiple FDA-approved drugs. What we produce today has a direct impact on the lives and well-being of patients around the world.”
Even though only two approved radiotherapies for cancer are on the market today, these treatment possibilities represent one of the fastest-growing fields in oncology research and testing.
What is a radiopharmaceutical?
Radiopharmaceuticals use highly targeted radiation to treat or diagnose a disease. To develop these therapies, a radioactive isotope, such as those produced at MURR, is linked to a targeting molecule that seeks out certain cellular features on a tumor. The isotope, linker and targeting molecule will form a radiopharmaceutical.
“The advancement of radiopharmaceuticals has created one of the most effective forms of precision medicine to date,” said Dylan Stoy, director of Therapeutic Strategy at PSI, a CRO with over a decade of experience working with these therapies. “With applications in diagnostics, therapeutics, or ideal theranostic pairings, radiopharmaceuticals give patients and providers a great option in an ever-growing list of cellular targets and indications — and I think we are just starting to see the tip of the iceberg.”
Leading-edge science and medicine meet when the radiopharmaceutical is administered to the body and sticks to cancer cells. The radioisotopes release energy that acts as a wrecking ball to cancer cells, eventually destroying them while leaving healthy cells unaffected, unlike the radiation therapies traditionally used in cancer treatment. As a result of this advanced treatment, used either on its own or in combination with existing therapies, patients typically face fewer side effects and have greater opportunities to resume a vibrant and healthy life.
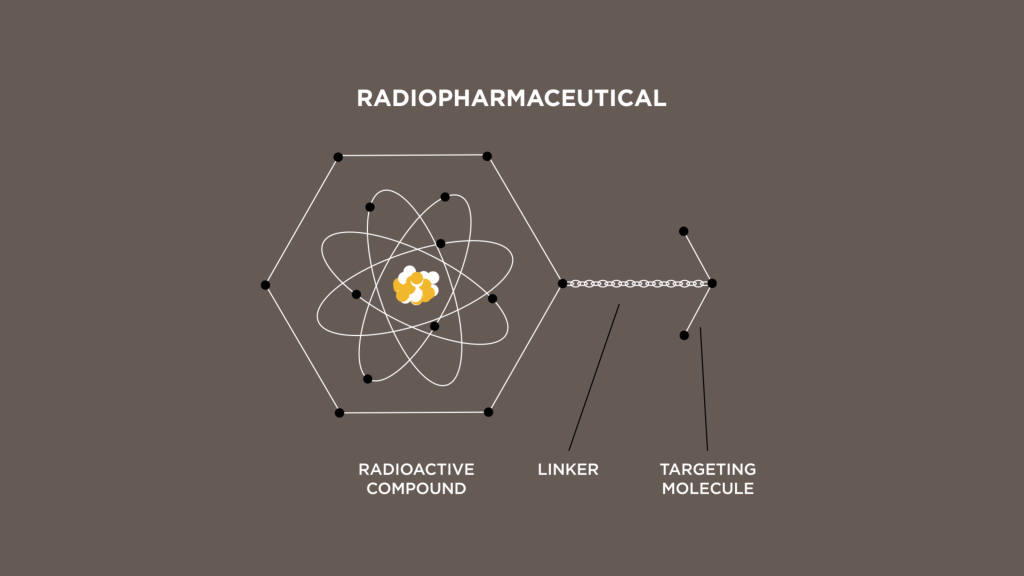
Making radioisotopes
The singular design, power and operating schedule of MURR allow the irradiation of stable isotopes to create the desired active pharmaceutical ingredient for treatments.
The center of the reactor has the highest concentration of neutrons, and these neutrons change less than one percent of a sample of ytterbium-176 to ytterbium-177 (Yb-177). Through radioactive decay, Yb-177 becomes Lu-177 in a matter of hours. A chemical process is then carried out under the FDA’s good manufacturing practices (GMP) to separate the lutetium from the ytterbium.
After the GMP process is complete, the active pharmaceutical ingredient — Lu-177 — is shipped to a pharmaceutical company for inclusion in its drug. With a half-life of 6.6 days, the entire process is designed to ensure radioisotopes produced this week will be administered to patients next week.
The long road of clinical research
Researchers join a radioisotope such as Lu-177 with two other components: a targeting molecule, which will guide the radioisotope to a specific cancer cell, and a linker, which connects the two. Each combination is tested to determine its viability. Once proven in the lab, the treatment is ready for clinical trials.
Although the timeline is lengthy and the results are not guaranteed, research and clinical trials to identify new radiopharmaceuticals are ongoing. These studies require highly specialized expertise and face many challenges — something Stoy and PSI know well. Today, PSI manages 50% of the industry’s pivotal radiopharmaceutical studies, which generate the data for drug approvals by regulatory authorities around the world.
“As the space continues to grow, it will be important to work closely with regulators across the globe to ensure reasonable access and availability,” Stoy said. “Although mapping for these products has improved significantly throughout the course of development, there are still challenges. In addition, many regulatory authorities, including the European Medicines Agency and FDA, are now working with groups dedicated to ensuring the safety of these treatments, meaning that an in-depth understanding of each specific country’s requirements is essential.”
This can be a challenge for large global studies like those PSI runs, as can the complexities of transporting radioactive materials such as Lu-177 with very short half-lives across borders in time to reach patients. Only a few nuclear pharmacies can provide the needed expertise and support for such trials.
In such cases, it’s often passionate individuals who drive this important research forward. PSI has built strong relationships around the world with more than 1,000 clinical research sites with experienced nuclear medicine teams. It has even created specialized roles to help overcome common delays during the clinical trial process. Reliable sources of manufacturing, such as MURR, will be the keystone to scalability as the field continues to progress.
Looking to the future
The success of drugs like Lutathera® and Pluvicto® has opened the door for new treatments, and researchers and pharmaceutical companies are looking at different radioisotopes and radiopharmaceuticals that can be used to treat and diagnose other types of cancer as well as diseases such as neurodegenerative disorders. Potential isotopes include terbium-161, actinium-225, lead-212, among others.
The demand for radioisotopes is rapidly increasing, and the University of Missouri is meeting the challenge. The university plans to build a new, larger, state-of-the-art reactor — NextGen MURR — which will expand the university’s capacity to produce medical isotopes that will be used in advanced cancer medicines for decades to come.
Co-authors on this article are Jared Hager and Dylan Stoy with PSI-CRO.